Introduction of AWHs for SOWS
Why is Sustainable Water Supply Needed?
As it has been reported [1], ~1.7 million deaths per year are caused by poor water quality, sanitation, and hygiene.
On the other hand, freshwater availability is essential for economic development in small communities in remote areas, where annual rainfall is negligible and traditional water sources such as rivers, lakes, wells, and springs are also not viable.
Moreover, in recent years the frequency and intensity of natural disasters induced by climate change have been dramatically increasing.
​
One of the priorities after a disaster is to provide safe drinking water. In the past, bottled water and/or water tanks were delivered to the affected population. However, limited water capacity and relatively expensive continuous delivery are the major drawbacks.
[1] Joule, 2, 1452–1475, (2018)
Sustainable Onsite Water Supply (SOWS) Technology
This Sustainable Onsite Water Supply (SOWS) technology is more practical and promising. There are two techniques to be usually applied to collect water: (1) desalination of the saline water (ground or underground water) and (2) extraction of water from the air. The source or SOWS of the water is usually selected based on availability and cost.
​
Current water desalination methods include (a) desalination, (b) dew evaporation, (c) reverse osmosis, (d) electrodialysis, and (e) electrosorption. However, for small-scale applications, these techniques both require accessible brackish water sources and expert labor for operation and maintenance. These requirements narrow the application conditions.
What is Atmospheric Water Harvester (AWH)?
The extraction technology of water from the air is called Atmospheric Water Harvester (AWH) or Atmospheric Water Generator (AWG).
​
Instead of other technologies, AWH is accessible everywhere and can be easily co-operated with a renewable energy source (e.g., solar energy) for local needs. Thus, SOWS technology is more practical and promising.
Generally, any viable AWH technology must satisfy five primary criteria [1]: it should be (1) efficient, (2) cheap, (3) scalable, (4) wide-band, and (5) stable enough to operate for a whole year or at least last a monsoon season.
Efficient
Cheap
Scalable
Wide-band
Stable
Currently, none of the existing commercial AWHs meet all these five criteria, because most of the AWHs are energy inefficient in terms of thermodynamics.
[1] Joule, 2, 1452–1475, (2018)
Work Flow of AWHs
AWH approach becomes a promising strategy for decentralized water production, to overcome the issues of long-distance transport or delivery of bottled water in remote areas [2].
The workflow of one AWH is usually described as follows: (1) capture the moisture from thin air, then (2) condense the captured moisture into liquid water.
[2] ACS Materials Lett. 2020, 2, 7, 671–684
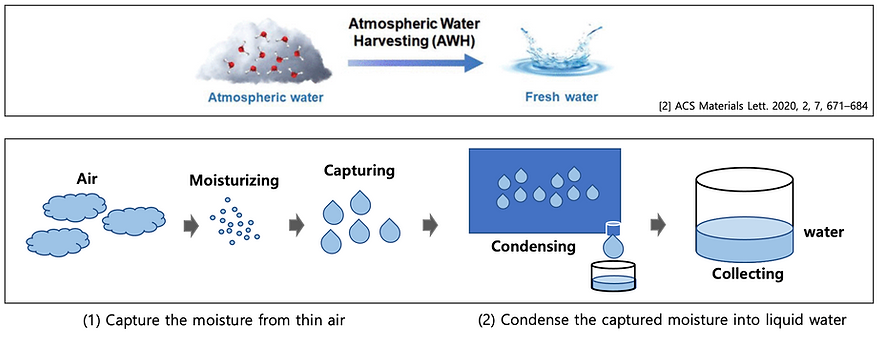
Performances of AWHs
Three indexes are usually used to evaluate the performance of an Atmospheric Water Generator, which includes:
1. The specific water production per day per unit collector area (SWP)
2. The specific energy consumption per unit mass water production (SEC), and
3. The recovery ratio of the feed air (RR).
​
1. SWP: evaluates the water productivity of the passive AWGs (no need for an energy input)
2. SEC and RR: assess the energy efficiency and the water vapor condensing effectiveness of the active AWGs (requires the energy input)

Ti and Di are the temperature and humidity ratio of the inlet air of the condenser, respectively.
Tcond represents the condensation temperature.
mH2O is the water production per unit mass of dry air (kg/kg).
εT and​ εd are the condenser's heat-exchange effectiveness and mass-exchange effectiveness, respectively.
The total cooling load of the moist air, Qcond, is the sum of the sensible heat load, associated with the temperature change of the moist air, and the latent heat load, associated with the enthalpy of condensation, is hfg
SEC x MHI = hfg : Moisture Harvesting Index [MHI]​
A smaller sensible heat load may result in a smaller SEC, which means a higher relative humidity of the inlet air is expected.
Categories of AWHs
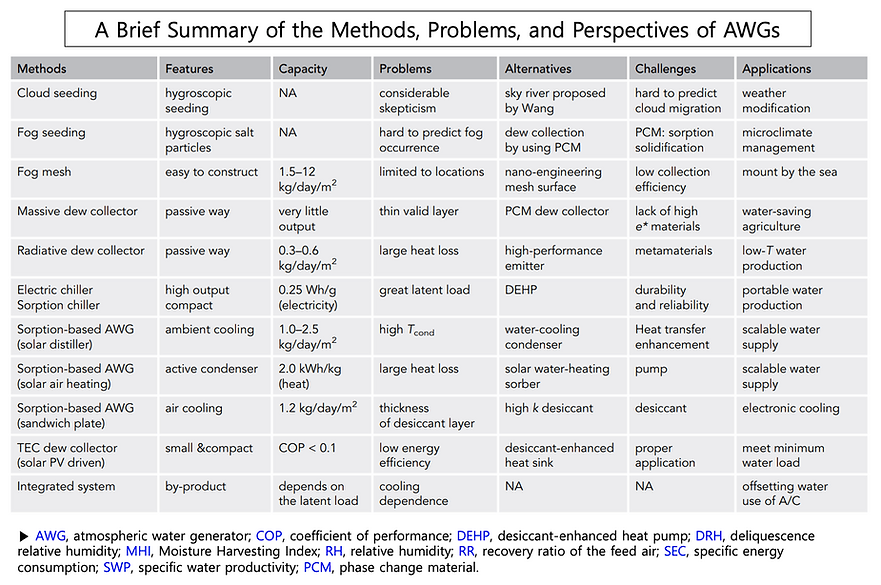
[1] Joule, 2, 1452–1475, (2018)
Hurdles of Materializing Sorption-based AWHs
Several remaining hurdles must be surmounted prior to the practical application of sorption-based AWHs with active condensers for scalable atmospheric water harvesting.
​
1. Lack of cost-effective heat sinks to maximize the SWP (specific water production per day per unit collector area)
2. Energy-inefficient ways applied to desorb the desiccants
3. Unreasonable design that fails to reduce heat loss or to achieve heat recovery before and after the condensing process
4. Ignorance of the kinetics in terms of material selection and absorber design
[2] ACS Materials Lett. 2020, 2, 7, 671–684
Outlook and Perspective Opportunities for AWHs
1. The chemical stability toward moisture (water) is necessary to eliminate the contamination of obtained water.
2. The interaction between materials and water molecules should be strong enough to enable the operation at low RH (relative humidity) or high-temperature conditions.
3. The concentrated moisture can be released by relatively small energy input.
4. The microstructure of moisture harvesters should be tailored to allow airflow passing through at high flux.
5. The structual strength is required to be strong enough to maintain the designed structure during the water release.
Enabling moisture as a water resource → one of the most promising alternative strategies to overcome the challenges of long distance portable water transport or delivery in water-scarcity areas[2].
[2] ACS Materials Lett. 2020, 2, 7, 671–684