Summary of published papers
(1) Recent advances in atmosphere water harvesting: Design principle, materials, devices, and applications
B. Wang et al., Nano Today 40, 101283 (2021). 10.1016/j.nantod.2021.101283
2021-12 upload
I. Introduction
In nature, many organisms survive and propagate in the extreme drought environment because they own their unique water harvesting skills. Understanding these strategies and functions of water harvesting skills in plants and animals can efficiently guide us to mimic and develop high-efficient materials and devices for water harvesting. → The critical principle and strategy in developing water harvesting materials include water capture/ condensation, water coalescence, and water transportation.
​
In this review, we summarize the latest progress in developing novel water harvesting materials via the rational design of the structure and surface chemical composition.
(1) These water harvesting processes' mechanisms and influence factors are introduced.
(2) Based on the developed materials, the water harvesting devices and systems are presented, including the non-energy consuming systems, solar energy-powered systems, and other external energy-consuming systems.
(3) The emerging water harvesting systems for agricultural applications are also reviewed.
(4) Finally, this review discusses the existing problems, challenges, and future research orientations in water harvesting materials, devices and their high potential in sustainable water supply in water-deficient regions, and agricultural irrigation systems.
​
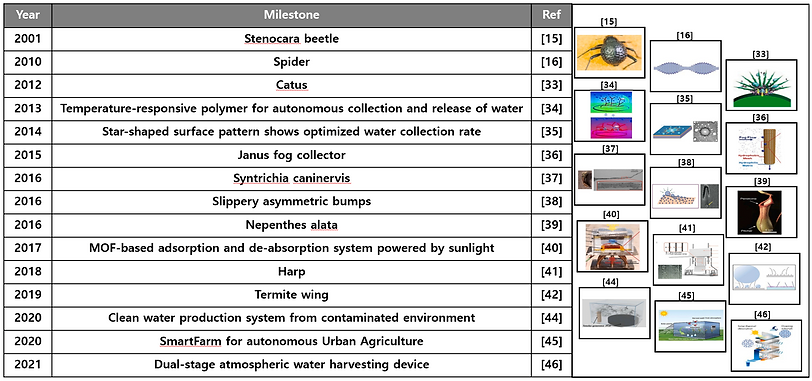
Timeline of the milestones of the bioinspired water harvesting
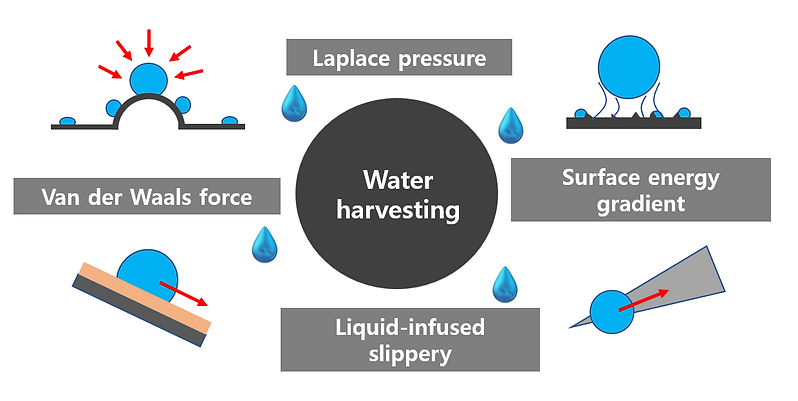
The mechanisms and driving forces for efficient water harvesting from the atmosphere
II. How to design high-efficient water harvesting material?
Key stages of the water harvesting process, mainly including four aspects: fog capture, droplet coalescence, directional transportation, and collection/storage.
​
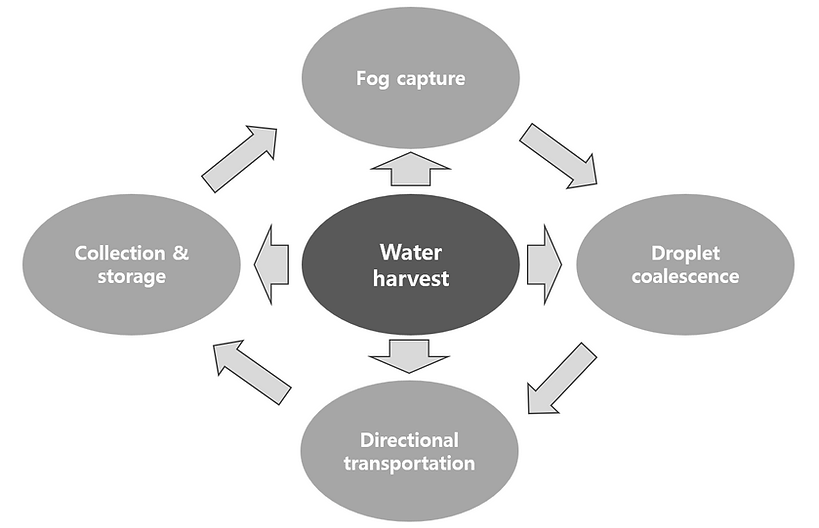
The mechanisms and driving forces for efficient water harvesting from the atmosphere
II-a. Environmental effects
The water harvesting efficiency of a material is related to both the water vapor content and the physicochemical property of the materials.
The high RH in favor of the harvesting of water, and even some daily things, such as glass, can condense the water droplets at an ultrahigh RH. → the existence form and the size of the water in the air can also affect the water harvesting ability of a material.
The liquid phase collection → (1) raindrop collection, (2) dew water collection, and (3) fog collection
The gas phase collection → it is much more difficult to be captured by a material. à The special design of material structure and composition may be required.

The capture of water with various scales by the structures with different sizes range.
II-b. Directional transportation
To design high-efficient water harvesting materials, → two aspects should be considered: (1) the capture ability of the materials and (2) the directional transportation of the captured and coalesced droplets.
The solid generally utilizes the Van der Waals force to anchor the water molecules approaching the surface.
Water molecules condense quickly while the surface is terminated with polar and hydrophilic groups, and water molecules condense very slow while the surface is terminated with nonpolar and hydrophobic groups.
The hybrid surface with both hydrophilic and hydrophobic terminal groups can possess comparable condensation ability of water molecules if the two types of groups are well arranged in proportion.
​
Directional transportation of water enables the continuous collection of water from the air.
→ Most of the transport processes of water are driven by simple gravity. However, gravity based water transportation, in some cases, will result in low collection efficiency because the surface in-plane direction can be hardly utilized for water capture and transportation
The capillary effect offers an important transport method to overcome the gravity force.
(a) Asymmetric wetting properties on a porous surface can directionally transport the water droplets from the hydrophobic face to the hydrophilic face due to the surface energy difference between the Janus materials [1].
(b) The self-pumped surface contains the hydrophobic surface, the hydrophilic absorber, and the tiny pillar patterns.
The tiny pillars capture and condense the fogs from the air. Directional transportation and absorption of the water droplets towards the hydrophilic absorber can be spontaneously performed while the droplets reach a threshold size [2].
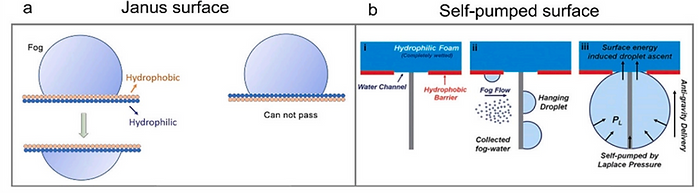
[1] Chemical Engineering J 383 (2020) 123168 [2] J. Mater. Chem. A 6 (2018) 20966
(c) The conical spine can capture fogs and directionally transport the water droplets under the synergy of conical morphology and wettability gradient along the cactus spine towards the root site [3].
(d,e) The slippery asymmetric bumpy surface is another optimized design that can condense the water droplets on the bumps and guide the transportation of water along the asymmetric slope [4,5].

[3] Nat. Commun. 3 (2012) 1247 [4] Nature 531 (2016) 78–82

[5] Nature 532 (2016) 85–89
(f) Inspired from the pine needle (S. chinensis), asymmetric (half-flat, half-curved) pillars can cause the directional flipping of droplets (droplets rotate from flat to the curved side of pillar). Furtherly, the height gradients of the tilted surface facilitate the long-range, and ultrafast transport of fog droplets [6].
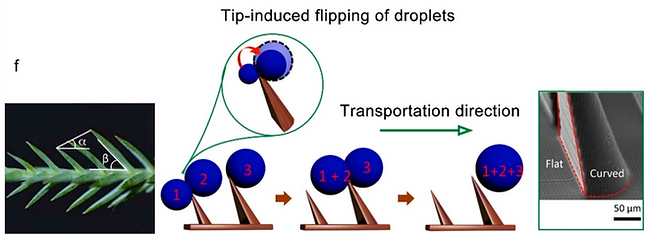
[6] Sci. Adv. 6 (2020) eabb4540
II-c. Design principles of adsorbent materials
Water harvesting by the adsorbent materials mainly involves the fog capture and absorption, and water desorption and condensation in a cyclical way.
Currently, solar and wind energy are sustainable and low-cost candidates because of their renewable feature, and are commonly used to drive the desorption process.
To amplify the fog capture capability, the surface may be constructed with a porous and structured surface with a large specific surface area..
For the adsorption stage at night-time, the environment is expected to be humid with relatively low temperature to obtain a large amount of water capture and storage. For the desorption stage at day-time, the environment is expected to be dry with a high temperature on the adsorbent material, and a relatively low temperature on the condenser.
II-d. Water harvesting materials development
II-d-1. Beetle back-inspired materials
Two decades ago, Parker and Lawrence discovered the survival skills of the Namib Desert beetle [7]. →
(a) The droplets from the fog laden wind accumulate on the hydrophilic bumps until they reach a critical size and roll down the hydrophobic valleys toward the mouth of the beetle [8].
The hydrophilic-hydrophobic contrast → for the purpose of developing water harvesting materials on various substrates → such as textile, cellulose film, composite mesh, copper mesh/sheet, stainless steel mesh, and so on.
(b) The bumped surface with periodical peaks → enhanced performance in water harvesting, because the water capture and coalescence process are shortened, and the re-evaporation of the microdroplets is reduced [9].
[7] Nature 414 (2001) 33
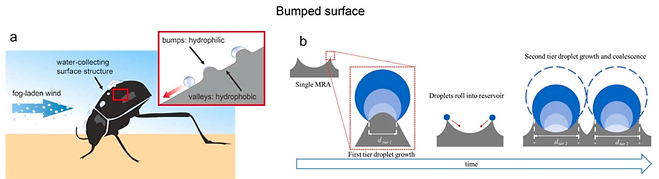
[8] Langmuir 34 (2018) 2933, [9] Langmuir 34 (2018) 13409
(c,d) The nanostructured surfaces with different pattern shapes, including the pure superhydrophilic surface, superhydrophobic surface, à circle shaped pattern, and star-shaped pattern [10].

[10] Sci. Adv. 6 (2020) eabb4540
(e) A kind of tree-shaped and branched hierarchical cones on a superhydrophobic film to achieve high-efficiency water harvesting with unique water transport direction [11].
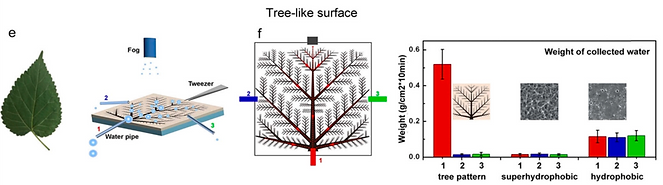
[11] ACS Appl. Mater. Interfaces 9 (2017) 29248
II-d-2. Cactus-inspired materials
Cactus species possess excellent skill in fog capture and directional transportation of water droplets under the synergy of conical morphology and wettability gradient along the cactus spine [12].
(a) The water capture and transportation efficiency of a cone structure are highly dependent on the apex angle, the surface wettability of the conical spine [13].
(b) Water collecting efficiency → the orientation angle of the barb should be backward facing instead of forward-facing [13].
(c) While the water droplets spontaneously transport to the root of the conical spine, one can apply a Janus and porous membrane (made of hydrophobic face and hydrophilic face) to allow the unidirectional penetration of the membrane. The penetrated droplets will fall into a container due to gravity [14].
(d) Another water collecting strategy, the water superhydrophilic absorbing sponge is installed at the root of the conical spine for continuous water storage [15].
(e,f) Scale-up of the cactus-inspired conical water collector → densely aligned conical spines can be installed both outside the curved spherical surface or inside the spherical surface [16].

[12] Langmuir 32 (2016) 9335 [13] Adv. Mater. Technol. 4 (2019) 1900727
[14] Small 14 (2018) 1801335. [15] J. Colloid Interface Sci. 530 (2018) 274
[16] Adv. Funct. Mater. 24 (2014) 3235
II-e. Adsorbent materials
Usually, water is taken up during the colder nighttime when the relative humidity is higher and desorbed during daytime. The necessary heat for desorption is ideally provided by solar radiation and requires no additional energy input [17].
At the micro-level, the temperature difference causes the structural change of the temperature-responsive polymer combined with a high surface roughness, which induces the reversible switching of the materials between the superhydrophobic state and superhydrophilic state.

[17] ACS Cent. Sci. 5 (2019) 1699
Researchers have paid intensive attention to the development of other kinds of absorption materials for tackling the water shortage from the perspectives of low energy consumption and ease of materials processing, including the hydrogels, 3D porous frameworks, textiles (b) [18], nanoscale powders (c) [19], and silica fibers (d) [20].
super moisture-absorbent gel, which integrates the hygroscopic chloride-doped polypyrrole (PPy-Cl) and the poly(N-isopropyl acrylamide) (poly-NIPAM) with switchable hydrophilicity (e) [21].
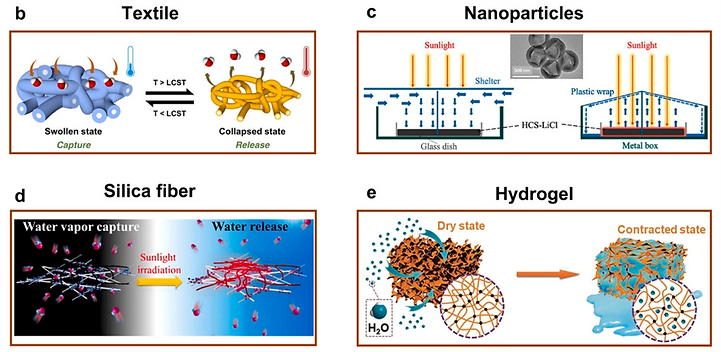
[18] ACS Sustainable Chem. Eng. 2019, 7, 24, 19870, [19] Nano Energy 67 (2020) 104255,
[20] Environ. Sci. Technol. 52 (2018) 53, [21] Adv. Mater. 31 (2019) 1806446
The adsorbent materials can not only harvest water for people’s drinking demand but also show their capability to adjust in-door humidity through adsorption and desorption processes and passive thermal management materials for surface cooling through the desorption process of water inside materials. The properties and application scenarios of the water harvesting materials are summarized in the following Table.
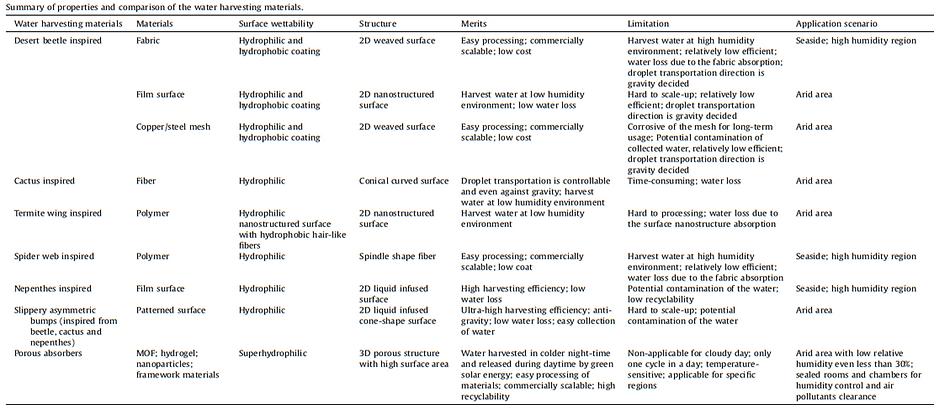
II-f. Device configuration design
II-f-1. Non-energy consuming systems
Many water harvesting systems have been constructed without external energy consumption, mainly in the form of interlaced meshes.
Compared with the meshes (which contain both the vertical and horizontal wires), they demonstrated that the fog harps could maximumly avoid the clogging issue whereas maintaining the high capture efficiency if the size and spacing of the fog harps are rationally designed [22].
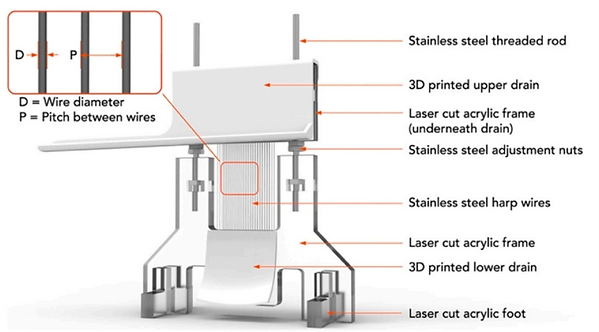
[22] ACS Appl. Mater. Interfaces 10 (2018) 11979
Fogwater harvesting system (FogQuest) contains 600 square meters of fog nets [23].
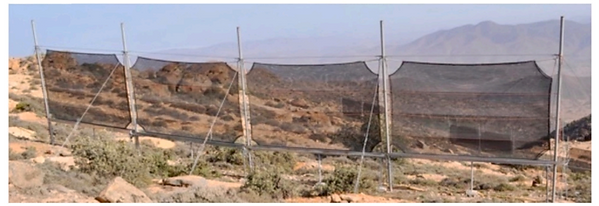
[23] Procedia Eng. 107 (2015) 186–193
II-f-2. Solar energy powered systems
Solar energy-powered devices generally utilize the natural variations of temperature and relative humidity between daytime and nighttime to absorb and de-absorb water in a recyclable manner.
The interfacial solar-heating-assisted atmospheric water generator (AWG) is based on salt-resistant GO-based aerogel. Water vapor sorption occurs at nighttime, and desorption occurs during the daytime under sunlight.

[22] Angew. Chem. Int. Ed. 58 (2019) 12054–12058
Solar energy-powered devices generally utilize the natural variations of temperature and relative humidity between daytime and nighttime to absorb and de-absorb water in a recyclable manner.
The materials capture/ absorb water during the nighttime and de-absorb water during the daytime.
→ To facilitate the water absorption effect during the nighttime, the materials can also be mixed/doped with hygroscopic materials, such as CaCl2. To promote the photothermal effect during the daytime, the materials are commonly doped/coated with heat-absorbing materials, such as graphene, polydopamine, etc. For example, graphene and graphene oxide have special photothermal effects and have been applied in water-absorbing materials for rapid water evaporation and collection.
​
For the adsorbent materials, high porosity and large specific surface area, broad light absorption range is indispensable. In addition, shaping the sorbents into forms suitable for easy processing, and storage, on the premise of maintaining their high adsorption feature and low cost is important.
​
For the device/system optimization, most of the devices are sealed or semisealed system that includes water condenser, heat sink, and reservoir. How to optimize the sorption-desorption cycles, and match it with the unpredictable weather condition is crucial for the high water yield.
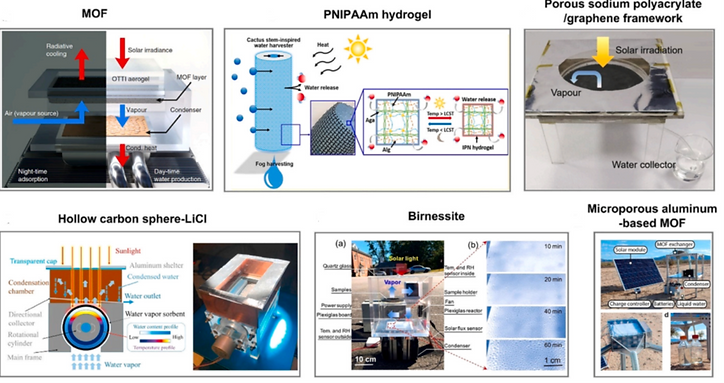
[23] Nat. Commun. 9 (2018) 1191, [24] ACS Sustain. Chem. Eng. 7 (2019) 10561,
[25] Adv. Mater. 32 (2020) 1905875, [26] Nano Energy 67 (2020) 104255,
[27] Environ. Sci. Technol. Lett. 7 (2020) 48, [28] ACS Cent. Sci. 5 (2019) 1699–1706
II-f-3. Solar energy powered systems
Apart from solar energy, other types of energy are proposed to actuate the water harvesting process. Generally, there is a trade-off between the input energy and the water production. For example, passive water harvesting may depend on radiative cooling and non-tunable condensation, resulting in limited water production and highly-sensitive efficiency to climatic conditions. While the other energy is supplied to perform active condensation, people can significantly enhance water production under various climatic conditions.
Schematic and a photograph showing the thermoelectric condensation platform. The successive images show the water condensation process [29].
Optical image and schematic showing the device used for centrifugal-force-assisted fog collecting [30].
Schematic showing the design concept of the water-collecting windmill inspired by rice leaf, cactus, and pitcher plant. The right insets show the schematic and SEM images of the blade [31].

[29] ACS Appl. Mater. Interfaces 10 (2018) 26759, [30] ACS Appl. Mater. Interfaces 8 (2016) 10005,
[30] ACS Appl. Mater. Interfaces 11 (2019) 17952
III. Prospects for application
The applications of the atmosphere water harvesting technology mainly focus on the drinking water production for humans in remote and arid regions, and agricultural irrigation of the plants and poultry in sustainable manner.
AirDrop, as a typical commercial water-harvesting device, is composed of a solar panel, turbine, hollow copper condenser, and water storage tank.
As water is the origin of all living things on the earth, water harvesting materials and devices show their high potential in agricultural application.
An automated and self-affordable solar-powered SmartFarm device that is capable of harvesting atmosphere water for irrigation purposes.
​
Self-affordable solar-powered SmartFarm device [31].
Schematic showing the water adsorption on the Cu-complex surface with one Cl vacancy.
→ exhibits a maximum water uptake of up to 300% and a water production rate of 2.24 g/g h−1 under sunlight irradiation.

[31] Adv. Mater. 32 (2020) 2002936
Photograph showing the SmartFarm consisted of Cu-complex, control panel, timer, motors PTFE sheet, and solar panels. The optical images at the bottom showing the wall of the SmartFarm at different times during the water release (left).
The time-lapse showing the growth of Ipomoea aquatica in the SmartFarm device (right).

IV. Conclusion and outlook
Summary of the water harvesting efficiency of typical materials.

Both the practical environment and the material design affect the harvested water. For design of a water harvesting material/surface, we may
(1) enhance the water collection efficiency by the increase of the capability of fog capture of a material,
(2) promote of the directional transportation,
(3) inhibit the evaporation of collected water,
(4) improve the sorption capacity of porous framework,
(5) lower down the desorption temperature,
(6) increase the light spectrum absorption for solarthermal sorbent,
(7) take the scale-up capability, biotoxicity and cost into account,
(1) The capability of fog capture of a material can be improved by construction of rough surface and surface grafting of stable molecules terminated with polar and hydrophilic groups.
(2) The promotion of the directional transportation to the reservoir may be enabled by the rational design of the surface topography and wettability. The conical spine, self-pumped surface, slippery asymmetric bumpy surface, and chemical gradient surface, are demonstrated to be helpful in the directional droplet transportation and collection.
(3) Inhibition of water re-evaporation is also a quite important part during the long-time harvesting process. One may apply the sealed and semi-sealed system to minimize the evaporation, the reduction of the environmental temperature to inhibit the evaporation, and the rationally designed condenser to converge the water.
â–¶ The future water harvesting systems may be also developed for agricultural applications, such that the water feeding of poultry, and irrigation of plants are able to be completed in an intelligent and self-affordable fashion. The water harvesting system also shows its capability on adjustment of in-door and in-cabin humidity through adsorption and desorption, which reveal its high potential applications in confined spaces such as spaceships and submarines.
(2) A Roadmap to Sorption-Based Atmospheric Water Harvesting: From Molecular Sorption Mechanism to Sorbent Design and System Optimization
K. Yang et al., Environ. Sci. & Tech. 55, 6542 (2021). 10.1021/acs.est.1c00257
2022-01 upload
I. Introduction
This review aims to summarize the latest progress in this field and provide perspectives for the further development of sorption-based atmospheric water harvesting (SAWH), focusing on the design of sorbent materials and the optimization of the entire system.
SAWHs are categorized into specific groups: (1) nanoporous solids, (2) hygroscopic polymers, (3) salt-based composites, and (4) liquid sorbents.
​
The workflow of SAWH is following.
(1) The moisture sorbent with a high affinity to water molecules (i) captures atmospheric water molecules and (ii) enriches them on the surface or within the internal structure.
(2) Once heated, (iii) the concentrated water vapor will be released from the sorbent, and then (iv) condensed and (v) collected as liquid water. After water release, the sorbents can be regenerated and reused in the next.
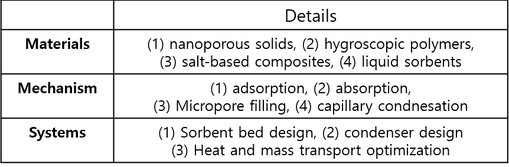
II. COMMON MECHANISMS OF WATER SORPTION
The fundamental mechanisms of water molecule capturing include (1) surface water adsorption, (2) micropore filling, (3) capillary condensation, and (4) absorption.
​
Adsorption is an exothermic process in which the adsorbate molecules accumulate on the adsorbent's surface. Introducing functional groups that can form hydrogen bonds with water molecules is a standard method to improve the hydrophilicity of the adsorbent surface.
​
When the adsorbent contains a porous structure, the adsorption occurs not only on the surface but also in the void space of the pores. (diameter ≤2 nm) The van der Waals potential of the pore walls overlaps, resulting in a much higher adsorption potential than that on the open surface.
Some sorbents, such as hygroscopic salts and ionic polymers, uptake water by absorption. Unlike adsorption, which occurs on the surface or in the pores of the sorbents, absorption is the incorporation of molecules into the bulk volume of the sorbents, which involves an initial surface capture step and a subsequent internal permeation process.
​
Nanoporous solids contain uniform (sub)nanosized pores that render large surface areas and pore volumes in the materials. When used for water adsorption, their well-defined pore structure gives rise to a significant change in water uptake under certain RH conditions, depending on the pore size and surface property.
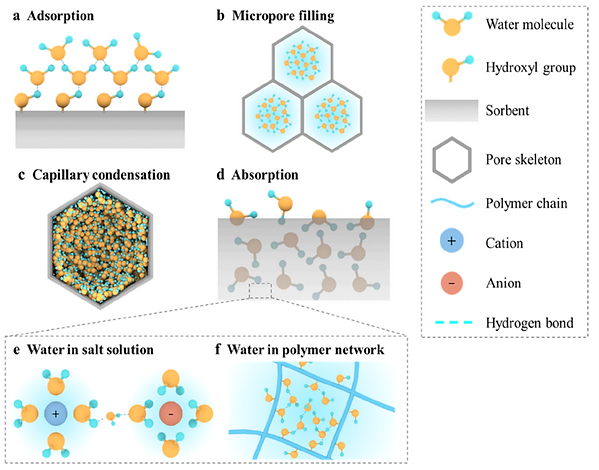
Schematic illustrations of different water sorption mechanisms
III. CURRENT DEVELOPMENTS OF MOISTURE SORBENTS
Ideal SAWH sorbents should have the following properties:
(1) high water sorption capacity under operational conditions, (2) fast kinetics to increase the number of sorption-desorption cycles within a certain period, (3) easy regeneration using low-grade energy, (4) stable performance in long-term applications.
​
III-a. Nanoporous Solids
Traditional nanoporous solids, such as activated carbons, silica gel and zeolites, can be used for water adsorption,
→ But they are not suitable for SAWH: (1) activated carbons are hydrophobic and contain a large proportion of inaccessible ultramicropores, so they exhibit low water uptake under common conditions, (2) silica gels also have limited water adsorption capacities, due to their low total pore volume, (3) zeolites require high temperature to release water from the highly hydrophilic micropores.
More scientific attentions have been paid on the development and utilization of novel nanoporous solids, such as metal-organic frameworks (MOFs), covalent organic frameworks (COFs), and aluminophosphate, to improve the water harvesting performance.
​
The effect of different organic linker modifications in CAU-10 on its water adsorption property, as reflected in the water adsorption isotherms (left of below figure) [1].
Water adsorption−desorption isotherms of MOF-801[2], Co2Cl2(BDTT)[2], and Cr-soc-MOF-154[4] collected at 25 °C. (right of the below figure)
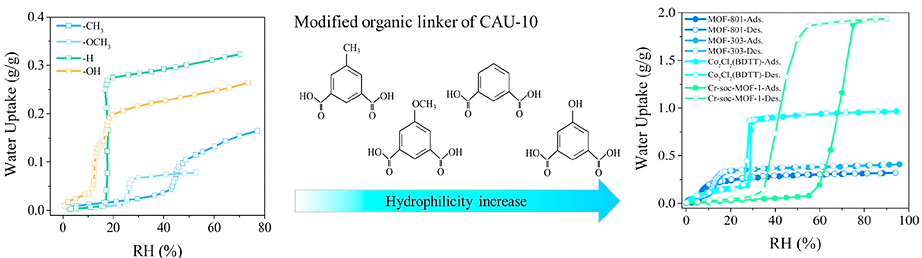
[1] Chem. Mater. 2013, 25 (1), 17
[2] Sci. Adv. 2018, 4 (6), No. T3198
[3] ACS Cent. Sci. 2017, 3 (6), 668−672
[4] Chem. 2018, 4 (1), 94−105
III-b. Hygroscopic Polymers
Unlike porous solids that capture water by adsorption using surfaces and porosity, hygroscopic polymers collect the atmospheric water by absorption, storing the captured water within the polymeric networks.
A recently developed hydrogel (hydrogel is a cross-linked, hydrophilic polymer) shows an ultrahigh water uptake capacity (4.20 g/g) when placed in an environment with RH > 90% [5], and it can release water at moderate temperatures (>55 °C) using sunlight as the energy source.
​
Researchers pay particular attention to their mechanical properties because the penetration of water molecules in polymer networks can cause structural expansion and affect the overall stability of polymers. → To improve the mechanical stability of sorbents,
(1) double network method, (2) dual physicochemical linking, and (3) nanocomposite incorporation have been developed [6].
[5] Adv. Mater. 2019, 31 (10), 1806730
[6] Chem. Rev. 2020, 120 (15), 7642

A porous hygroscopic aerogel (PGF) composed of sodium polyacrylate (PAAS) and graphene for solar-driven SAWH, [7] Adv. Mater. 2020, 32 (6), 1905875

Thermal-responsive hydrogel for moisture uptake and liquefied water release,
[8] Adv. Mater. 2019, 31 (10), 1806446
III-c. Salt-Based Composites
Hygroscopic salts are low cost but highly effective desiccants. Deliquescent salts such as CaCl2, LiCl, and LiBr are able to absorb water molecules for 1~3 times of their own weight at medium RH conditions → They have gained widespread attention in the field of SAWH.
However, one drawback limiting their usage is that they will eventually dissolve in the collected water, resulting in (1) reduced absorption kinetics, (2) system corrosion, and (3) difficulty in recovering the sorbents. → To address this issue, the most popular strategy is to incorporate the deliquescent salt into a designed matrix. Loaded salt plays a major role in capturing water molecules while the matrix acts as a container to hold the finally liquefied salt.
The reported matrices can be classified into (1) porous materials, (2) hollow structure containers, (3) fibrous substrates, and (4) polymer networks.
(1) Silica gels, mesoporous silicates, and porous carbons are widely used matrices for deliquescent salts. → Compared with these traditional porous materials, some MOFs have much larger pore volume, and thus higher loading capacity.
(2) Using hollow containers with large internal space is an effective strategy to raise the upper limit of salt loading content → A mesoporous hollow SiO2 sphere (HS) (diameter ∼100 μm) and nanoscale hollow carbon sphere (HCS).
(3) Capillary forces generated from the micro gaps between the fibers can hold the liquefied salt to limit the salt leakage to a certain extent. → There are relatively few related studies about SAWH at present.
(4) Hydrophilic PAM hydrogel is flexible and can hold the liquefied salt inside its hydrophilic cross-linked network making the pore volume no longer a limiting factor for the loading capacity. à Above 70 °C to fulfill efficient water release using sunlight.

Fabrication of deliquescent salt-based composite sorbents using different matrix materials
III-d. Liquid Sorbents
Liquid desiccants provide a level of equilibrium water vapor pressure lower than free water displays at the same temperature [9],
à thereby absorbing water from the surrounding air.
Common liquid desiccants include two types: (1) concentrated salt solutions (LiCl, CaCl2, and LiBr) and (2) ionic liquids (ILs).
The hygroscopicity of ILs mainly depends on the type of cation and anion, as well as their interaction strength. : Ac− > Cl− > Br− > TFA− > NO3− > TFO− > BF4− > Tf2N− > CHO− > PF6−. Liquid desiccants have the advantages of system continuity and low regeneration temperature (40-70 °C)
​
Left) Water sorption occurs in the night time when the RH is relatively high, and the desorption process is triggered by sunlight in the day time → As the evaporation proceeds, evaporation enthalpy will increase with the solution concentration, leading to a decreased evaporation rate.
Right) To address this issue, a simultaneous adsorption-desorption mode has been proposed, in which an additional adsorption region is designed to continuously collect water and then transport the captured water to the desorption region via diffusion.
[9] Energy and Built Environment 2020, 1 (1), 106
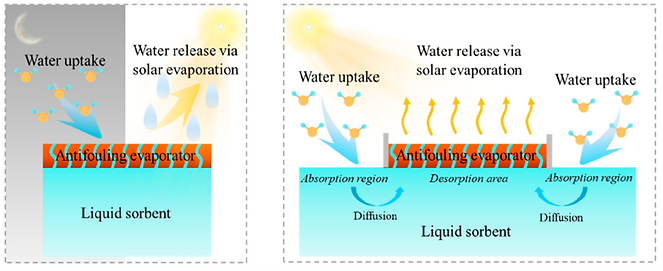
Interfacial heating-assisted atmospheric water harvesting
A modified water harvester that allows a simultaneous adsorption−desorption working mode
IV. SYSTEM-LEVEL DESIGN STRATEGIES FOR A SAWH HARVESTER
USorption-based atmospheric water harvesters share the same basic working principles: (1) moisture enrichment on/in the sorbent, (2) release of water vapor from the sorbent by heating, (3) liquefaction of water vapor on the condenser.
​
IV-a. Enhancement of Mass Transport and Heat Transport in Sorbent Bed
Without external forces, the transport of water molecules is realized by spontaneous migration along the humidity gradient.
For the temperature-actuated water harvesting cycle, particular attention should be paid on the heat transfer. In fact, mass transfer and heat transfer affect and interact each other. For example, compaction of sorbents can increase the thermal contact, but the resulting low porosity will hinder the interparticle vapor transport and vice versa. Therefore, these two aspects should be carefully balanced.
To reduce the thermal resistance, two effective strategies can be employed. (1) The first one is to minimize the heat transport path by fabricating a thin layer of sorbents. (2) The second approach is to increase the thermal conductivity by incorporating with highly thermal conductive materials.
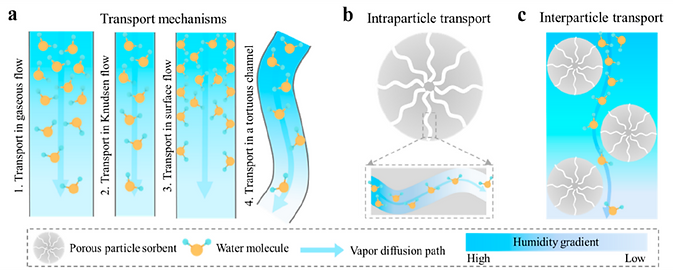
(a) Different mechanisms for the transport of water molecules in porous media.
(b) Intraparticle transport of water molecules in the sorbent.
(c) Interparticle transport of water molecules in the sorbent bed.
IV-b. Liquefaction Enhancement of the Condenser
In most SAWH systems, the water vapors released from the sorbent bed are directed to a condenser where they are liquefied for collection. This process is usually conducted in a closed environment, and it can be described by the psychrometric chart
During desorption, the captured water is released from the sorbents by heating, causing the surrounding air to be heated and humidified (Point I to Point II). Driven by the vapor pressure gradient, the released water vapor diffuses to the cold condenser and transfer heat to the condenser through conduction to cool down (Point II to Point III). Once reaching the dew point, water vapor is condensed for collection (Point III to Point IV).

For example, in order to buffer the released condensation heat and prevent the condenser temperature from rising significantly, condensers with high heat capacity are preferred.
The temperature fluctuation between day and night can also be employed (right figure). Specifically, cold storage material (e.g., water) can store the cooling energy at night when the temperature is relatively low, while in the daytime, it can serve as a low-temperature condenser for efficient vapor condensation.
Apart from these passive condensation processes, active cooling technology (e.g., thermoelectric cooler) can also be used for condensation enhancement, although it suffers from the disadvantage of requiring high-grade energy input such as electricity.
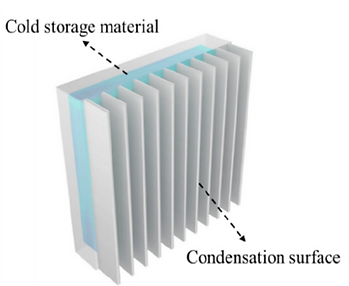
In addition to controlling the condenser temperature, the liquefied water should be rapidly removed, otherwise the formed water layer will act as a thermal barrier (with a low heat conductivity of 0.6 W/m/K) to hinder or even stop the subsequent liquefaction.
Vapor condensation goes through nucleation, droplet growth and then droplets coalescence. Unfortunately, conventional condenser surfaces, whether hydrophilic or hydrophobic, can hardly realize fast nucleation and rapid droplet removal at the same time.
A hydrophilic surface may assist the nucleation, its high retention to water also retards the water droplets removal.
Hydrophobic surfaces allow condensed droplets to roll off by gravity when the size of water droplet increase to be comparable to the capillary length (∼27 mm); however, high energy barrier of the hydrophobic surface is not conducive to initial nucleation. Additionally, it is still difficult to fabricate a large defect-free hydrophobic surface. As a result, droplets will attached to the defects on the surface, impeding the heat transfer.
​
Namib Desert beetle can use its special droplet-producing system on its back exoskeleton, where bumps with hydrophilic top and wax-covered hydrophobic slope are randomly distributed. On the surface with mixed wettability → water vapor nucleate on the hydrophilic top with low energy barrier and can roll off easily through the hydrophobic path after the water drop reaches the critical size.
It has been found that bumpy patterns with mixed wettability is more efficient than plain surface for droplets nucleation and collection [10]. By mimicking the bumpy patterns with rationally designed wettability, many advanced condenser surfaces have been fabricated, offering vast potentials for the condensation enhancement in SAWH.
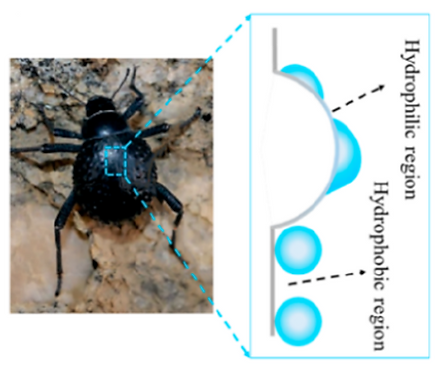
[10] Nature 2016, 531 (7592), 78
Jumping droplets is a unique phenomenon occurring on nanostructured superhydrophobic surface [11], where water droplet (∼10−100 μm) can spontaneously jump out of the plane due to the release of excess surface energy upon small droplets coalescence.
To enhance the overall hydrophobicity and make droplets to roll off easily, this kind of surface is usually designed to be rough, having knifelike microstructures, pyramidal-like micro prominences or micro textures similar to these kinds.
As illustrated in right-hand side, (1) water vapor nucleates and grows into droplets within rationally designed microstructures; once (2) two neighboring droplets coalesce, (3) the merged droplet will be driven away from the microstructure by the released surface energy and (4) roll off along the hydrophobic surface. Such a droplets jumping mechanism offers an efficient avenue for the removal of condensed water from the condenser.
[11] Phys. Rev. Lett. 2009, 103 (18), 184501

IV-c. Design of Various Water Harvesters
Ithe evolution and classification of these practical water harvesters
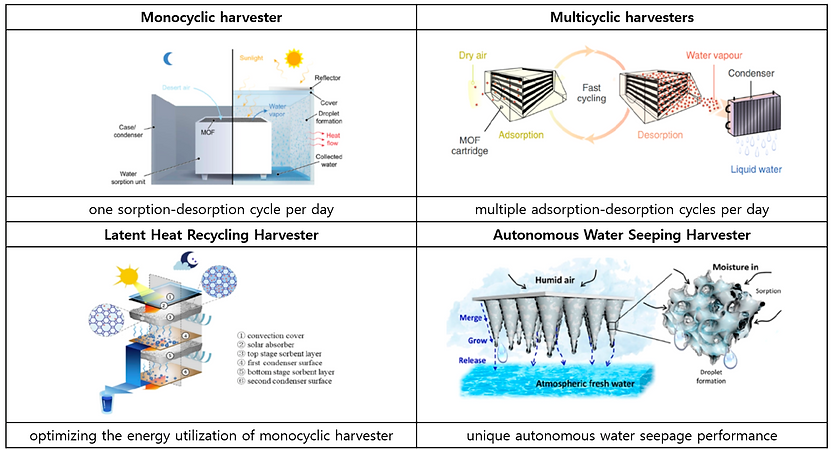
(3) Mimicking bumpy patterns with mixed wettability of Namib Desert beetle (Summary of previous publication)
2022-07 upload
I. Introduction
Namib Desert beetle can use its special droplet-producing system on its back exoskeleton, where bumps with hydrophilic top and wax-covered hydrophobic slope are randomly distributed. On the surface with mixed wettability, water vapor nucleate on the hydrophilic top with low energy barrier and can roll off easily through the hydrophobic path after the water drop reaches the critical size.
It has been found that bumpy patterns with mixed wettability is more efficient than plain surface for droplets nucleation and collection. By mimicking the bumpy patterns with rationally designed wettability, many advanced condenser surfaces have been fabricated, offering vast potentials for the condensation enhancement in SAWH.

II. Summary of previous publications
There are many attempts and studies to mimic the structure of bumpy patterns with mixed wettability to collect water efficiently from the fog and dew. Here some previous publications are summarized in the following Table.
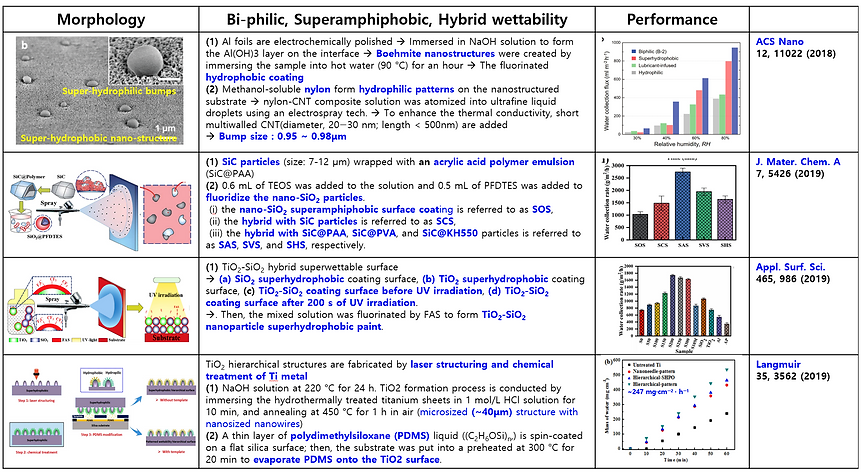
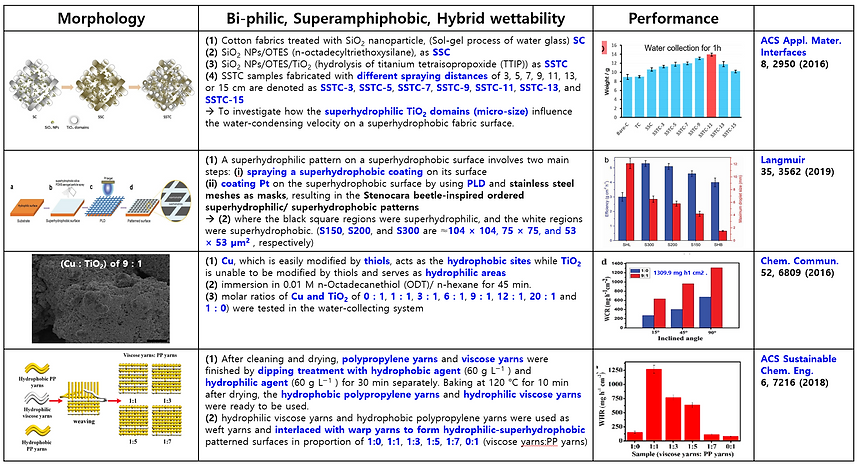
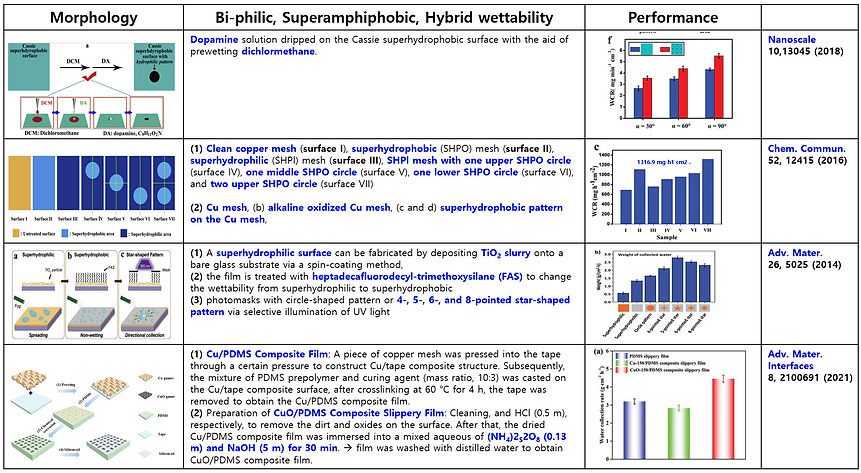

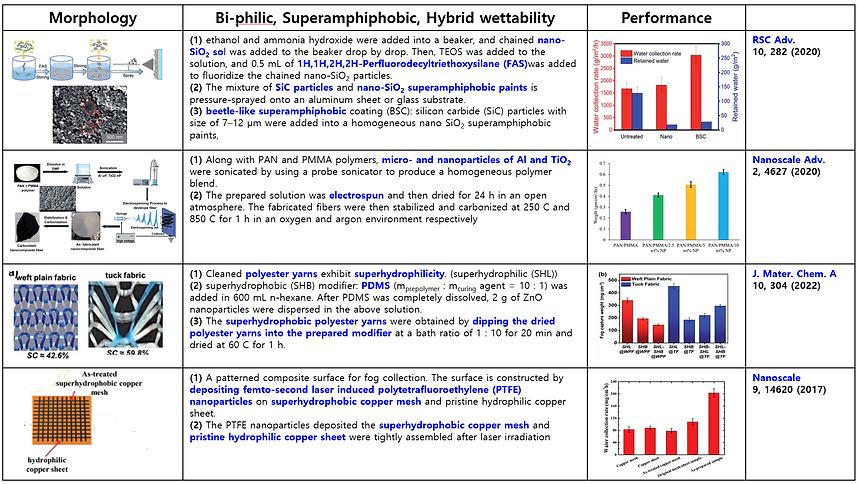
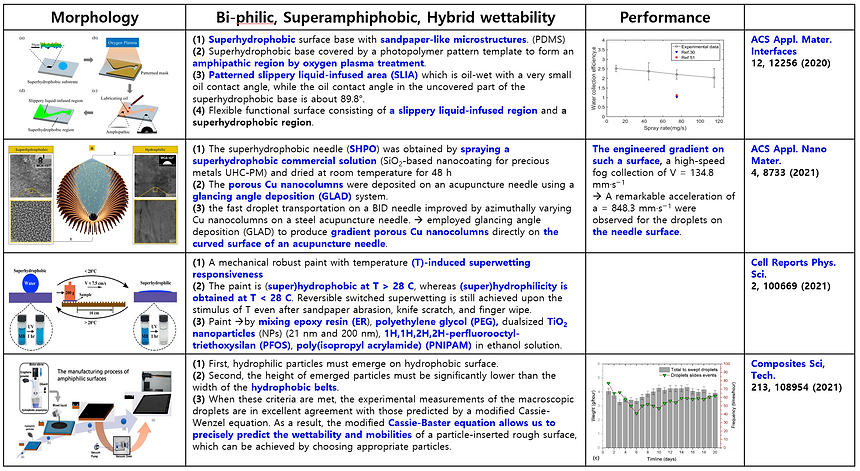
(4) Energy performance and climate dependency of technologies for fresh water production from atmospheric water vapour
R. Peeters et al., Environ. Sci.: Water Res. Technol., 6, 2016 (2020) 10.1039/d0ew00128g
2022-12 upload
I. Introduction
The share of withdrawn water returned to the source is dependent on the application.
There are three ways to resolve local fresh water shortage.
First, when the supply from local fresh water sources is insufficient, water transportation over long distances from areas with water abundance is an obvious solution.
Second, seawater is an inexhaustible water source. Desalination of seawater and transportation with pipelines inland is an example of this solution.
Finally, an important observation is that atmospheric water is largely sufficient to cover the human fresh water needs.
​
Estimated global water distribution [1].

[1] in Water in Crisis A Guide to the World's Fresh Water Resources, ed., Oxford Univ. Press, 1993, pp. 13–24
Water withdrawal in different sectors split into a consumed and returned fraction in billion metric ton (bmt) in 2014 and a forecast for 2040. Data extracted from the IEA (2016) [2].

[2] IEA, Water- Energy Nexus, 2016
In this review a thermodynamic analysis of the energy requirement of water production from air is provided. The boundary conditions depending on geographical location can vary substantially and the variability of the water content of atmospheric air and climatological conditions are part of the analysis.
Implementing water-from-air technology would have a large impact on the water-energy nexus
II. Parameters of water vapour in atmospheric air
The relative humidity (Φ) is the ratio of the partial pressure of water vapour (Pw) over the saturation pressure (Ps) (eqn (1)).

The dew-point (Td) is the temperature at the onset of condensation and at which the relative humidity reaches 100%.
The absolute humidity (ω) at different relative humidity (Φ) levels is plotted against temperature in the below figure. The upper curve at 100% relative humidity is the saturation curve where condensation occurs. Unsaturated air can reach saturation by cooling or by humidification [3].
​
[3] Thermodynamics an engineering approach, McGraw-Hill, 5th edn, 2006
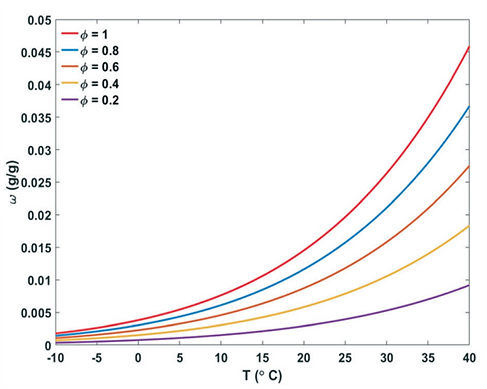
The mathematical relation between relative humidity (Φ), absolute humidity (ω), total air pressure (P) and temperature (T) is given in eqn (2).
​

The dew-point Td can be obtained from this equation by solving for T and setting Φ = 1 for a given absolute humidity and air pressure
​
The water vapour saturation pressure Ps at temperature T (°C) can be described mathematically with the empirical AERK Magnus formulation, such as e.g. in the range of T = [−40 °C, 50 °C] by eqn (3).

The total air pressure P is simply the sum of the partial pressure of dry air Pa and Pw, the vapour pressure of water in the air (eqn (4))

The total moist air enthalpy consists of a dry air term Ha and a water vapour term Hwv (eqn (5)).
The heat capacity of air Cp,a is taken as a constant of 1.005 kJ kg−1 C, which is a reasonable assumption in the temperature range [−10 °C, 50 °C]. The average heat capacity of water vapour Cp,wv in the interval [−10 °C, 50 °C] equals 1.82 kJ kg−1 C and the water vapour enthalpy at 0 °C is 2500.9 kJ kg

The heat of condensation of water (ΔHvap) at atmospheric pressure and normal boiling temperature (100 °C) equals 2257 kJ kg−1 , which is a large amount of energy. This large amount of energy has to be removed to enable the phase change of water from vapour to liquid.
III. Principles of water extraction from air
Atmospheric water generators can be divided into categories.
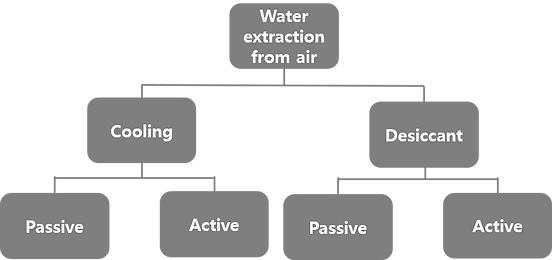
Water vapour can be extracted from the air either by (1) cooling and condensing moisture out of the air, or else, by (2) using a desiccant material.
The term passive indicates water is captured with the help of natural phenomena and without an energy delivery system. In the active case, an external energy vector is implemented to assist the water extraction process.
A theoretical performance evaluation will be based on the specific water yield SYwater (L kW−1 h−1 ) which represents the volume of liquid water which can be produced with an energy input of 1 kW h.
IV. Technologies of water extraction from air
IV-a. Passive cooling technologies
IV-a-1. Principle of dew formation
Passive radiative cooling of a condensation surface to a surface temperature below the dew-point leads to deposition of liquid water originating from water vapour in the air. à (for example) Namib beetle.
Any surface outdoor emits energy by radiation of electromagnetic waves in the infrared wavelength region to the sky (the below Figure) [4].
The available cooling power lies between 25 and 100 W m−2 on cloudless nights. à A theoretical maximum water production of 0.8 L m−2 per day under most optimal conditions has been estimated [5].
Consequently, a large surface area exposed to the sky is needed to collect artificial dew in significant amounts [5].
Air flow provides a larger flow of water vapour toward the cold surface, but depending on the temperature of the wind, it also can counteract the radiative cooling.
[4] Renewable Energy, 2018, 119, 662.
[5] J. Hydrol., 2008, 361, 159.

IV-a-2. Principle of dew formation
Fog consists of tiny water droplets suspended in the atmosphere.
→ These droplets nucleate homogeneously in saturated air.
→ Droplet nucleation is favoured by the presence of fine suspended particles acting as nucleation centre.
​
Fog collection is the capturing of the already existing liquid water droplets of air aerosol on a mesh material (the below Figure) [6].
The droplets grow by coalescence on the mesh and are finally detached from the mesh and collected by gravity.
The amount of fog that can be harvested depends on relative humidity, absolute humidity, wind velocity and the frequency of fog occurrence.
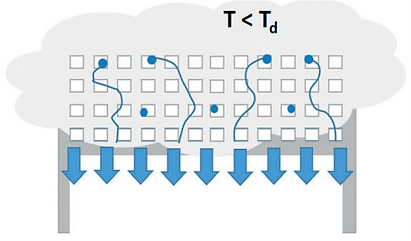
[6] J. Geophys. Res.: Biogeosci., 2007, 112, 1
IV-a-3. Dew and fog collection devices and applications
The performance of dew collection devices are available.
​
​
​
​
​
​
​
​
The performance of fog collection devices are available.


Conductive heat loss to the underground is an alternative way of cooling a condensation surface.
→ Air is forced to enter an underground metal coil connected to a reservoir for collecting the condensate.
→ The metal coil is in thermal contact to the underground.
→ The minimum required temperature difference between the underground and atmospheric air derived from the temperature dependence of the relative humidity is presented in the following Table.
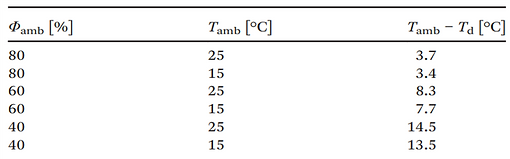
Air at T = 15–25 °C needs to be cooled by ca. 14 °C at Φ = 40%, 8 °C at Φ = 60% and 3.5 °C at Φ = 80% to initiate condensation.
Problem: The temperature difference between air and underground condensation surface vanishes rapid.
​
Temperature differences between atmospheric air at ground level and underground at 2 m deepness are available for a location in Lecce, Italy [14]. → The temperature difference shows seasonal fluctuations.
The temperature difference between air and underground reaches a maximum of about 8 °C in July.
→ Air at relative humidity below 60% will never reach saturation under these conditions.
→ At 80% relative humidity, temperature differences of 3.5 °C will only be reached in certain months.
→ Active cooling of the condensation coils appears to be needed to reach the desired temperature difference.
[14] Energies, 2014, 7, 84651
IV-b. Active cooling technologies
IV-b-1. Process description and thermodynamic analysis
In most cases, active air cooling is based on an evaporation–condensation cycle of a coolant to extract heat from a cold reservoir (evaporator of the coolant) and transportation of the extracted heat to a hot reservoir (condenser of the coolant).
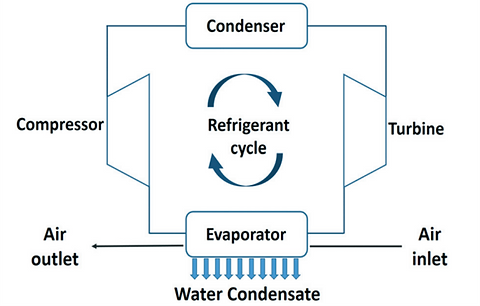
This water extraction concept is based on a reverse Carnot cycle of a coolant. The coefficient of performance of the cooling unit (COPcooling) is defined in the following eqn [15].
Tc the temperature of the cold reservoir
Th the temperature of the hot reservoir.
The hot reservoir is typically at ambient temperature, Tamb.
​
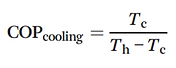
[15] Thermodynamics an engineering approach, McGraw-Hill, 5th edn, 2006
[16] Int. J. Water Resour. Arid Environ., 2011, 1, 142
Thermoelectric coolers (TEC) are an alternative to the evaporation–condensation cycle of a coolant [16].
→ This TEC forces heat transport by application of a voltage difference between a semiconductor pn-junction.
TEC based cooling processes are generally less energy efficient, but the absence of a recirculating fluid is a practical advantage.
The general process scheme of an active cooling device to condense water vapour out of air.
The theoretical maximum amount of liquid water that can be recovered from air for a given power supply in a 100% efficient process without heat recovery is given by SYwater,
The condensation temperature Tc has a strong impact on the specific water yield.

The condensation temperature Tc has a strong impact on the specific water yield.
​
A humid hot climate
The specific water yield under these conditions shows a clear maximum at 17 °C.
→ the maximum specific water yield (SYmaximum water), :21 L kW−1 h−1 .
→ When the ventilator power is omitted the optimum specific water yield is 32.6 L kW−1 h−1.
​
A desert climate
There is a shallow optimum energy efficiency around 3 °C.
→ The yield of liquid water per kW h is small and does not surpass 3.3 L kW−1 h−1.
→ The contribution of the ventilator power is negligible in this case.
The maximum specific water yield for a given climate condition was estimated at the corresponding optimal condensation temperature Tc,opt.

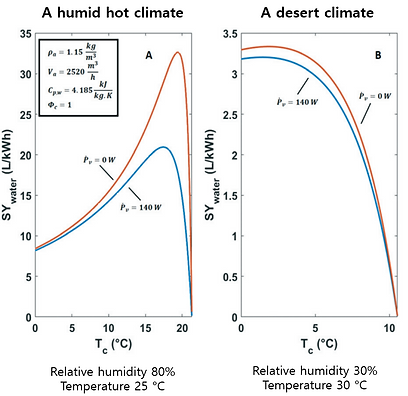
For relative humidity values of 20, 40, 60, 80 and 100%, the specific water yield at optimum condensation temperature is plotted against ambient temperature.
​
The higher the relative humidity, the higher the maximum specific water yield, and the more water can be condensed from air at a given ambient temperature.
Evidently, at 100% relative humidity, it takes little energy to produce dew on the collector surface.
The minimal ambient air temperature necessary for avoiding water freezing in the cooling section of the device at optimal energetic performance was estimated at 3, 7, 13 and 24 °C for relative humidity of 80, 60, 40, and 20%.

IV-b-2. Principle of dew formation
Water extraction from air is very common in air conditioning. Dehumidifiers based on the process of active air cooling to force condensation of water vapour contained in the air are established technology.
​
The efficiency of reported devices was estimated based on reported specific water yield and an estimated theoretical maximum.
​
​
​
The efficiency of commercial dehumidifiers is ca. 40%.
​
Portable self-filling water bottles are miniaturised versions of the air cooling and moisture condensation technique.
Where the moisture condenses onto t thermoelectric cooling surfaces.
A power input of 313 W is required to produce 0.5 L h−1 when only condensation heat needs to be removed and when taking a maximum COPcooling value of 1 for a TEC device.22,45,55
At full irradiation, a PV panel typically delivers 180 W m−2 , therefore around 1.7 m2 of solar panel is required to supply enough power. The specific water yield of TEC based air cooling is estimated to be in the range of 0.14–1.1 L kW−1 h−1, 55-58
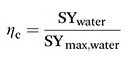
IV-b-3. Membrane assisted water condensation
This setup reduces the total cooling requirement by increasing the relative humidity of the inlet air of the condenser.
​
​
​
​
​
​
​
​
​
​
​
The specific water yield of the modelled membrane dehumidifier setup reached 6.18 L kW−1 h−1 with a 5000 m2 membrane surface area.
→ liquid membrane with hygroscopic salts on a carbon black nanoparticle
→ Polymer electrolyte membranes
​
In this process (i) a net water flux over the membrane is created by splitting water electrochemically into oxygen gas and protons at the anode side of the membrane and (ii) combining these protons again with the oxygen gas at the cathode side to make water. (iii) In addition, water moves through the membrane by the effect of electro-osmosis. Back diffusion lowers the performance of this set-up
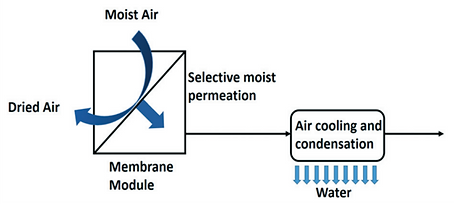
IV-b-4. Conclusion
Specific water yields are in the range 2–4 L kW−1 h−1 . Miniaturised portable systems exist, but are less energy efficient.
In dry climates the dew-point of humid air is below 0 °C which makes the cooling concept impractical because of the need of defrosting the condenser for collecting the harvested water.
​
The condensation temperature could be enhanced above freezing temperature by implementation of a membrane module to increase the relative humidity prior to condensation.
The membrane/condenser combination significantly improves the specific water yield. The reduction of energy consumption in the cooling unit can balance the additional investment in a membrane module.
IV-c. Passive desiccant technologies
Trapping water in solid and liquid desiccants is an alternative to condensation by cooling.
​
IV-c-1. Process description and thermodynamic analysis
The temperature difference between day and night can be used to conceive an adsorption–desorption cycle.

Night and day cycle of passive water extraction from air with a desiccant (grey spheres). Water adsorbs at night from cold air on desiccant material and desorbs by solar heating during the day. Water vapour desorbing from the adsorbent is condensed in a separate container [17].
The difference between both states (qads at Tads) and (qdes at Tdes) defines the extractable amount of water in a night and day cycle.
​
The determination of the water extraction capacity from adsorption and desorption branches of the adsorption isotherm are explained in the following figure.
The difference between both states (qads at Tads) and (qdes at Tdes) defines the extractable amount of water in a night and day cycle.
The adsorption isotherm of a desiccant at Tads and Tdes defines the suitability of an adsorbent to serve atmospheric water harvesting.
[17] Desalination, 1987, 67, 227–229
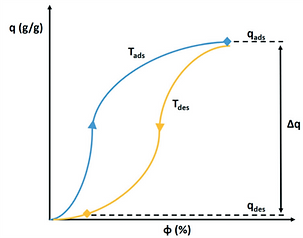
The heat of desorption is dependent on the desiccant material, temperature and uptake at which the process is operated, reflected in the adsorption isotherm.
The desorption temperature is selected in the range of 40–80 °C.
​
The specific water yield of a desiccant based process is defined as
The water production (Lh−1 ), equals the water volume captured during the night.
In the estimation of the total power supply (kW), the contribution of the sensible heat losses of heating the air itself and the desiccant mass are not included.
​
​
The estimated maximum specific water yield is plotted in the following figure against ambient temperature at desorption temperatures of 40, 60 and 80 °C, and neglecting ventilator energy input.
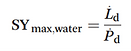
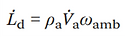




Maximum specific water yield of a passive solid desiccant based water-from-air extraction device against ambient temperature. The curves pertain to different desorption temperatures. (left)
Specific water yield of a passive solid desiccant based water from air extraction device against ambient temperature (Φ = 40%) at different heat of desorption values at Tdes = 60 °C. (right)
Using adsorbents with a lower heat of desorption enhances the specific water yield. Ventilator power requirements are negligible relative to the heat of desorption.
​
IV-c-2. Passive atmospheric water capturing devices with desiccants
Reports on water yields with passive devices using desiccants at different locations and climates are summarised in the below table. Literature typically reports water yields per surface area, or per weight of desiccant.
Harvesting potential of different hygroscopic materials

Estimates of specific water yields and efficiencies (the below eq.) were derived from the available literature data, and assuming the following parameter values for SYmax,water: ΔHdes = 3300 kJ L−1 , = 0 W, and no sensible heating.
​
​
​
The maximum specific water yield (SYmax,water) amounts to 1.09 L kW−1 h −1 . Note that this maximum and the actual energy efficiency will deviate because the desorption energy of the materials may be different from the assumed value.
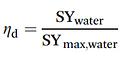

IV-c-3. Kinetics of water uptake by desiccants
Water uptake kinetics matter to the efficiency of the water collecting process. The adsorption step is found to be slower than desorption [18]. In addition, concentrating the solar heat can enhance desorption kinetics [19]. This makes the adsorption step the bottleneck of this kind of system.
​
The saturation process of typical adsorbent materials is illustrated at low relative humidity (Φ = 30%) in the left figure, and at high relative humidity (Φ = 90%) in the right figure. à It should be remarked that kinetic data from literature is difficult to compare because there are many experimental parameters involved, like ad- or absorbent bed geometry, particle size, temperature and pre-treatment conditions
[18] Nano Energy, 2020, 67, 104255
[19] Sol. Energy, 2018, 169, 302–315
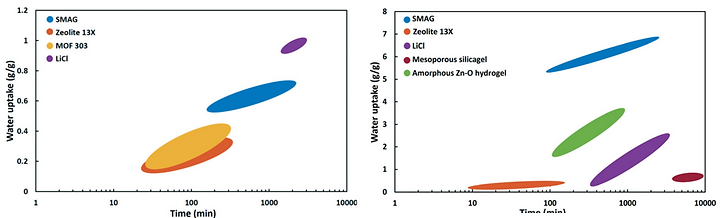
For example, silica gel is a well-known desiccant. It has high capacity, but the adsorption kinetics are extremely slow. ​→ After 10,000 minutes there is hardly 0.7 g g−1 adsorbed.
IV-c-4. Water recovery from desiccants
Ad- and absorbent materials have largely different regeneration requirements.
Regeneration temperatures and recovered water fraction for different desiccant materials.
​

Estimation of the maximum specific water yield when using liquid desiccants is more complicated than for a solid adsorbent.
IV-c-5. Conclusion
Passive desiccant based water harvesting is appealing because of its technical simplicity.
In recent years there has been innovation in desiccant materials, and especially MOFs and thermo-responsive polymers bear great promise.
IV-d Active desiccant technologies
Continuous water production is made possible by implementing an artificial energy supply.
​
IV-d-1 Process description of active desiccant technology
Continuous regeneration of the desiccant can be achieved by fixing the solid desiccant in a wheel.
→ Process flow diagram of the active desiccant technology based on the desiccant wheel design [20].

[20] A review of desiccant dehumidification technology, 1993
The rotating wheel moves the saturated solid desiccant to the regeneration section where the adsorbed water is evacuated by hot air. The desorbing moisture is collected by condensation in a downstream condenser.
A discontinuous process is another alternative.
The desiccant is used more efficiently in the active system, which reduces the desiccant material costs for a given water production rate.
IV-d-2. Active atmospheric water capturing devices with desiccants
One example of an active desiccant based device is a panel which produces drinking water from air, by using solar thermal energy and photovoltaic electricity.
Wang et al. reached an SYwater of 0.84 L kW −1 h −1 by using LiCl enclosed in an active carbon felt matrix as adsorbent and an electric heater for the discontinuous desorption stage [21].
The high efficiency can be explained by the humid conditions under which this excellent performance was achieved in combination with an efficient heater.
[21] Energy, 2017, 138, 542–551
IV-d-3. Conclusion
The use of ab- and adsorbent materials can be maximised by involving auxiliary energy.
That energy can be used to move the adsorbent physically from adsorption to desorption sections of the device, and to provide heat for regeneration.
Energetically, it does provide some benefits over the passive system mainly by the ability to use heat regeneration. The specific water yield amounts to 1 L kW −1 h −1 at the maximum.
V. Water from air and the water-energy nexus
From the analysis of the different concepts in this review, passive desiccant technologies and active cooling emerged as most promising technologies.
The climatological conditions favouring active cooling versus passive desiccant technology depending on relative humidity and ambient temperature are indicated in the below figure.
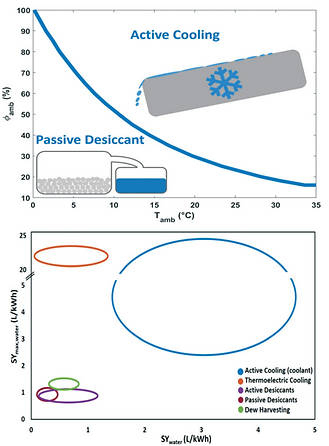
Optimal regions for active air cooling and passive desiccant technologies as a function of ambient temperature and relative humidity values, based on the theoretical maximum specific water yield.
Active cooling is the preferred choice in hot and wet climates.
In dry and hot regions especially vulnerable to water scarcity, desiccant technologies are the best and only choice when considering the atmosphere as a water source.
The maximum specific water yield and the achieved specific water yield of five different water-from-air technologies.
Relatively high specific water yields can only be reached with active cooling.
The limited productivity according to the state-of-the-art (∼1Lm−2), limits the application potential of passive desiccant water extraction to small communities.
​
Thermoelectric power plants consume on average 1.25 L kW −1 h −1 [22] which exceeds even the specific water yield to be expected
[22] Water Consumption Factors for Electricity Generation in the United States, 2016.
V. Conclusion
The maximum specific water yield (L kW −1 h −1) is a consistent parameter to evaluate water-from-air technologies and indicate the preferred climate conditions for their operation.
​
A continuous water output is achieved with the active cooling approach by using forced air convection over a condenser.
àIn this approach the maximum specific water yield decreases exponentially with decreasing relative humidity.
​
A more common approach in dry climates is the application of desiccant materials. → This led to the introduction of several new desiccant materials like MOFs which show lower desorption temperatures, improved adsorption kinetics and better applicability in extremely arid regions with a relative humidity around 30%.
​
There is plenty of water in the atmosphere, but harvesting it in an energy efficient way remains one of the grand scientific challenges. Introducing fundamentally new concepts may be key in making this technology a viable solution to combat water scarcity.
(5) MOF water harvester produces water from Death Valley desert air in ambient sunlight
Nat Water 1, 626–634 (2023). https://doi.org/10.1038/s44221-023-00103-7
2023-08 upload
I. Introduction
This paper highlights the global challenge of freshwater scarcity and the pressing need to address this issue due to various factors, including climate change, urbanization, and water pollution. Here are some key points from the passage:
Freshwater Scarcity: Despite Earth being called a "blue planet" because of its vast water reserves, accessible freshwater is quite rare. Only 2.5% of global water resources exist as freshwater, with much of it locked in ice or groundwater.
Growing Water Crisis: Currently, around 4 billion people live in water-deprived regions, and it is predicted that more than half of the global population will experience a water crisis in the future. This is a significant global challenge that affects numerous people.
United Nations Sustainable Development Goal 6 (SDG6): The passage mentions that providing a promising solution to the global water crisis is recognized as the United Nations' 6th Sustainable Development Goal (SDG6). This underscores the importance of addressing this issue on a worldwide scale.
Water Treatment Technologies: Efforts have been made to develop water treatment technologies such as seawater desalination and water purification to provide clean drinking water. These technologies have been effective in many regions but may not be suitable for arid, landlocked areas with poor economies.
Geographical Disparities: The passage also highlights the geographically non-uniform distribution of water resources. Like sub-Saharan Africa, arid regions and areas with poor economies often face significant challenges accessing clean drinking water, even with mature and large-scale water treatment technologies.
This paper underscores the importance of addressing the global water crisis, affecting billions worldwide. It also emphasizes the need for innovative solutions that consider geographical disparities and the unique challenges different regions face in securing clean and accessible drinking water.
The good news is that there is a lot of water in the air as vapor, which can be a handy water source. Instead of directly cooling the air (like dew or fog), we can use "sorption" to grab this water vapor, especially in dry places with low humidity.
Older ways of pulling water from the air could have been better. They used materials that did not suck up much water vapor and needed a lot of energy. But in 2017, a team led by Kim found a way to make a water harvester using a "metal-organic framework" (MOF) that can grab moisture even when the air is super dry, with humidity as low as 20%. Since then, there have been significant improvements in this method, thanks to better materials.
But there is still a problem: the designs of these water harvesters need to be more detailed. So, even with super materials like MOFs, they do not work well in arid deserts. For example, in 2019, Fathieh's work with MOF-801 produced little water - just 100 grams for every kilogram of MOF in one cycle. That could be better.
To make more water, most researchers must add extra energy. They either heat things a lot (using concentrated light or heaters) to extract water from absorbent or cool things down (using stuff like refrigeration or water cooling) to absorb moisture from the air.
​
​
II. Adsorption-Desorption-Condensation
Sure thing! Designing the device is essential for how well it works in real life. In Atmospheric Water Harvesting (AWH), there are three key stages: sorption, desorption, and condensation, and scientists need to design them carefully to get the most water.
In the sorption stage, which occurs at night when it is more humid, they anticipate that the sorbent material will quickly soak up much water at the proper humidity levels (as shown in the following figure). But when they use Atmospheric Water Harvesting (AWH) in real situations, it is essential to make lots of this sorbent material efficiently on a large scale. Some previous efforts have succeeded in making sorbents eco-friendly, but people should also carefully assess the costs and economics of these methods.
​
​
​
​
​
​
​
Desorption. When they get the water out of the sorbent (desorption), they must heat it using sunlight during the day. To keep that heat where it needs to be, they must ensure it does not escape into the air through conduction, convection, and radiation, as shown in the following figure.
​
​
​
​
​
​
​
Condensation. When turning water vapor into liquid (condensation), it is better if it happens at a lower temperature because it helps us collect more water. But to make this work well, we need to eliminate the heat from the condensation process, as shown in the following figure. In the past, people used active methods, like fans, to cool things down and get more water. They did this using electricity, which is acceptable in a lab but more challenging in remote desert areas where we only have sunlight. So, for places like deserts, people need to figure out how to do this without relying on electricity.
​
​
​
​
​
​
Heat and mass transfer. MOFs are the materials we use, and they usually need to be more packed together, with lots of tiny holes. This makes it tricky for heat to move through them when making the MOF bed.
To help heat move better, the primary fix is to mix MOFs with materials good at conducting heat, like metal foam or carbon-based stuff.
But here is the thing: when we pack the MOF crystals too tight in the bed, it can slow down how quickly they can soak up water. To make these harvesters on a large scale, we need to think about how we shape the sorbent material, make a structure with different levels, or increase the surface area where the air meets the material. All these things are connected, so people have got to think about them all together.
​
III. MOF-based AWH
This paper created a MOF water harvester that does not need extra power, just sunlight. It is great for getting lots of water from desert air and gives us an excellent example of making passive harvesters.
They used MOF-303, which is good at grabbing water and putting it on a special kind of foam with graphite. This foam helps spread heat around, as shown in the following figure. The MOF pellets they made keep their size even when they get heavier with water.
​
​
​
​
​
​
​
During the day, when the sun is shining, the heat makes the water in the MOF become vapor again. This vapor naturally moves to a different part because of temperature differences. To keep this heat from getting away, they put something like a vacuum seal around it. All these things together made the process work well. They noticed a 10°C temperature difference compared to a device without the vacuum, and the difference in temperature between the MOF and the condenser was as high as 35°C.
And the way they shaped the sorption part like a cylinder made it work well in real situations where the angle of the sunlight changes from morning to evening, as you can see in the following figure.
​
​
​
​
​
​
​
​
​
​
​
When designing the part that collects water (the condenser), people often use coatings that either attract moisture or repel it. The water-attracting surfaces make droplets form quickly while water-repelling surfaces help remove droplets.
In their work, they used a special coating made of CNT/PTFE (carbon nanotube and polytetrafluoroethylene, hydrophobic) on an aluminum surface (hydrophilic), as shown in the following figure. This coating was good at repelling water. It collected water faster and 45 minutes earlier than the regular aluminum surface. The water-harvesting capacity increased from 150 to 248 grams per kilogram of MOF.
​
​
​
​
​
What is cool is they tested this in Death Valley, a super dry place with hot daytime temperatures (up to 56°C) and very low humidity at night (as low as 14% relative humidity). Despite the harsh environment, they managed to collect water successfully in this high condensation temperature of 65°C.
Their tests showed that this setup could collect 210 grams of water per kilogram of MOF per day in Death Valley, twice as much as the previous passive MOF water harvester used in the Arizona desert, USA.
In summary, scientists in this paper made a device that can pull water directly from the air in a desert without needing extra power. They considered many things in making it work and got a lot of water - 210 grams for each kilogram of a unique material (MOF). They did this without using any extra energy in Death Valley, USA. This could be a helpful guide for designing future devices to fight water shortages.
But there is still more work before these devices can be used widely. Most studies look at how much water you can get for each kilogram of MOF, but they also need to know how much water the whole device can produce, which people need. They must figure out how to make these devices bigger, cheaper, and more efficient.
Plus, in actual weather, especially when it is cloudy or rainy, we might need more sunlight to make these devices work. So, we need to find ways to store energy for those times. That is a challenge, but technologies like batteries and keeping heat could help.
So, making passive Atmospheric Water Harvesting (AWH) a practical way to get clean drinking water is still a big job that needs the help of many different experts.
​
​
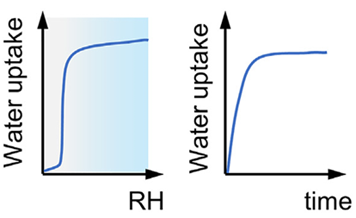
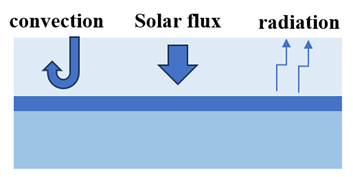
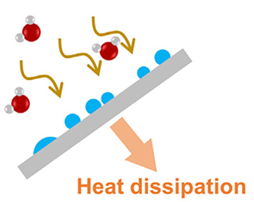

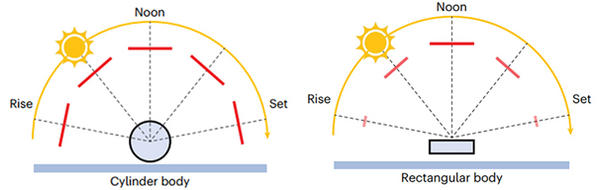
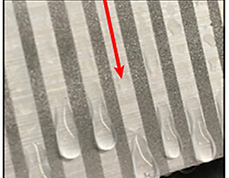